Quantum physics: the ‘laws of the absurd’, explained

Matter, when infinitesimal, behaves in a very eccentric way. This has been known for more than a century, and practical applications are now being explored. The world is on the verge of the second quantum revolution.
Absolutely everything that exists – you, me, the air we breathe, the leaves on the trees, my bike, your dog, the planet Jupiter, or the screen you are reading this on – is composed of molecules. Molecules are collections of atoms, which are in turn collections of protons, neutrons and electrons. If you look at them on this very, very small scale (something like 10-18 metres), you see that these particles, these “grains” of matter, behave very differently from the large objects they form.
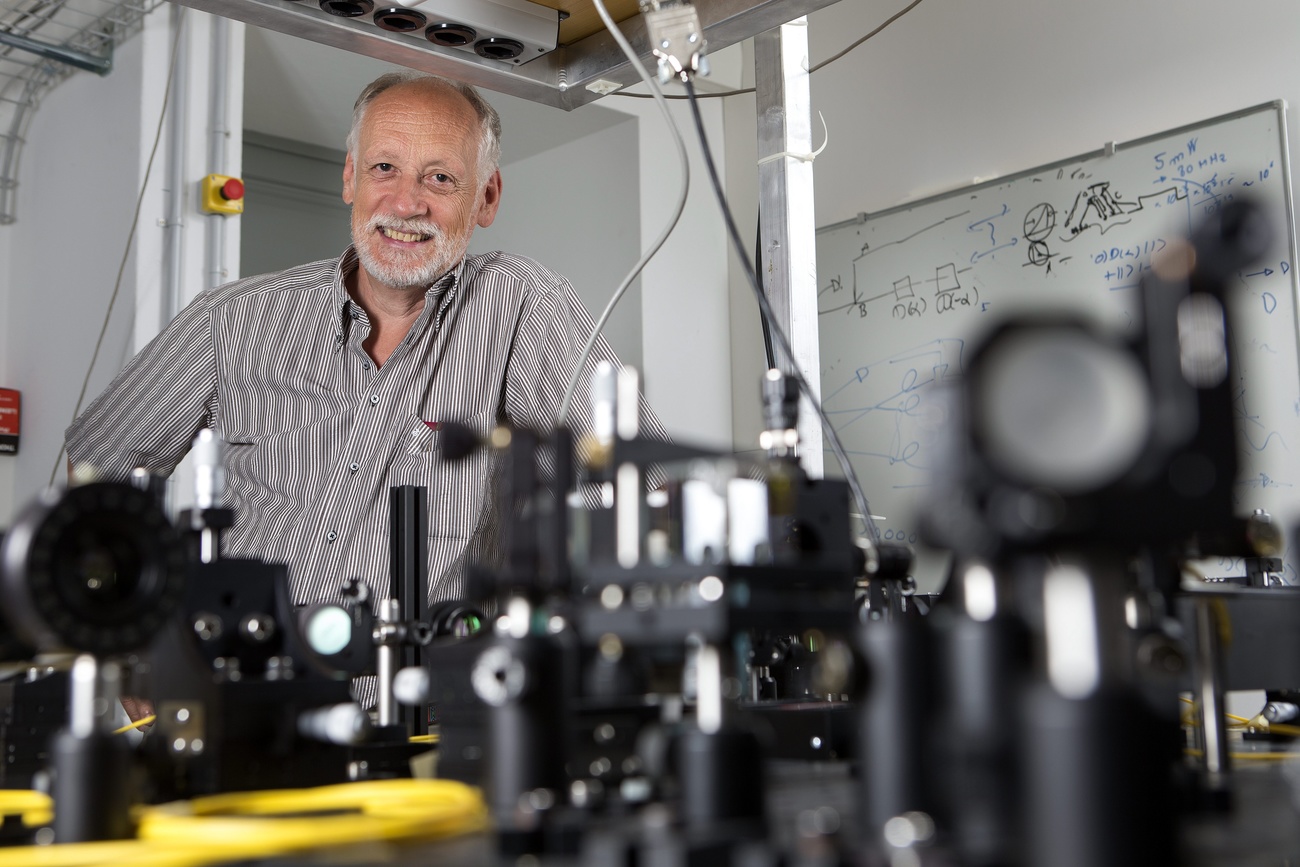
More
Quantum technology enjoys first commercial successes
An electron or a photon (“a grain of light”) can pass through two holes at once, be in several places simultaneously, or rotate in several directions at the same time. Stranger still, these particles seem to be able to communicate from one point in space to another, faster than light. They can even teleport!
“If quantum theory doesn’t shock you, it is because you haven’t understood it,” said Niels Bohr, who won the Nobel Prize for physics in 1922 and was the first to unite the various discoveries that gave rise to quantum theory.
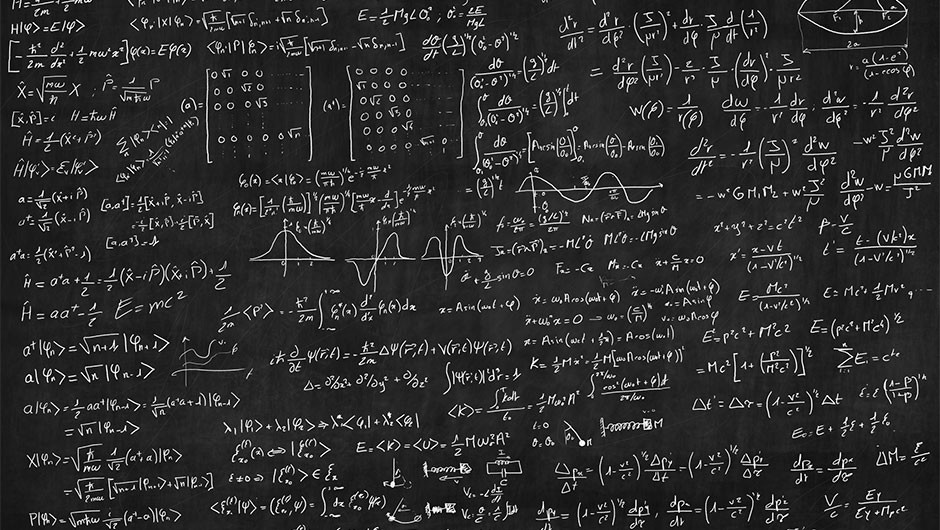
How have we known all this for so long, but no one has ever seen anything as small as an electron?
“The impressive power of human intelligence has managed to combine observation of nature with mathematical constructions,” said Nicolas Gisin, a physicist and director of the new Swiss Quantum CommissionExternal link, and author of a popular bookExternal link on the subject.
At the end of the 19th century, physicists were convinced that they had unlocked the last secrets of matter and expected no further advances in their discipline. But there were still some phenomena that conventional physics could not explain. For example, the way in which the colour of an object changes when it is heated. Under the flame of a blowtorch, a piece of metal changes from red to yellow, then to white, and then – in theory – it should emit ultraviolet light, which the human eye cannot detect. So it would be invisible. But this never happens, because in reality it emits far less ultraviolet than the theory predicts.
It was this anomaly that put Max Planck, winner of the Nobel Prize for physics in 1918, on the trail of a new theory that explains the behaviour of the infinitesimal. Rubbing his eyes and hoping to be mistaken, in 1900 Planck presented the hypothesis that energy (light is a form of energy) is not emitted continuously, but in the form of small packets, which he called quanta. Like water that flows in drops, instead of a continuous stream.
Albert Einstein, winner of the Nobel Prize in physics in 1921 and an employee of the Federal Patent Office in Bern, seized upon this discovery and in 1905 proposed his theory on the photoelectric effect. It assumes that light is not a wave, as previously believed, but a beam of particles, quanta, which were to become known as photons.
So is light a wave or a beam of small beads?
It is both. But also neither. The second generation of physicists at the beginning of the 20th century – Bohr, Louis de Broglie, Paul Dirac, Erwin Schrödinger, Wolfgang Pauli and Werner Heisenberg (all Nobel Prize winners) – showed that photons, electrons and other particles actually behave both as small grains of matter and as waves. This led Heisenberg to wonder whether it was “possible for nature to be as absurd as it seems?”
And what about Schrödinger’s famous cat, locked in a cage where it can be both dead and alive? The cage would have to be opened to find out whether the animal was dead or alive. Schrödinger proposed this purely theoretical experiment in 1935 to demonstrate that the quantum world is based on a sum of probabilities. That said, it would be feasible only if the cat were a particle, not a living being made up of billions of atoms.
Just like Planck (to start with) and Einstein, Schrödinger only got into quantum physics to demonstrate its shortcomings. However, he was eventually convinced. Einstein, on the other hand, was always reluctant to accept a theory that left so much to chance and was based on probabilities and statistics. For him, the universe was entirely decipherable, and “God does not play dice”, as he told Bohr in 1927.

Chance versus determinism, Planck versus Einstein: who was right, and who enabled the first quantum revolution?
Planck. And after him Bohr and his disciples, assembled in what was called the Copenhagen School. As confusing as it seems, and as uncomfortable as it is for students who have to set aside everything they have previously learnt and approach it with a fresh mind, quantum theory has never been disproven.
It has enabled us to understand how atoms work and, to a very large extent, how they bind together to form molecules, which has opened the way to spectacular advances in chemistry and biology. In technology, it is the understanding of quantum mechanisms that has allowed us to control the flow of particles (electrons or photons) that drive our lasers, radios, televisions, computers and mobile phones. All these objects that appeared in the second half of the 20th century are already quantum technologies.
What can we expect from the second quantum revolution?
In 2022 the Nobel Prize in physics was once again awarded to theorists in the field of quantum physics. Alain Aspect, John F. Clauser and Anton Zeilinger have all worked on what is known as entanglement.
This is one of the most puzzling properties of particles. When two are entangled and you change the state of one, the other will take the same value. Instantly and even if it is at the other end of the galaxy. “This remains an absolute mystery. It’s as if these correlations appeared from outside space time,” Nicolas Gisin told us in 2008, after a quantum teleportation experiment carried out at the University of Geneva.
Teleportation? Yes. About 20 years ago we entered the second quantum revolution. Now we no longer manipulate flows of electrons or photons but these same particles (and even atoms) individually. Quantum cryptography and other “simple” applications have already entered everyday life, but the holy grail remains the quantum computer. By using the very special properties of quantum particles, it would theoretically be endowed with computing power beyond the reach of conventional machines.
We’re talking about very complex operations which would make it possible to model new drugs or new materials, to optimise distribution networks, batteries or solar cells, to understand the mechanisms of photosynthesis, and much more.
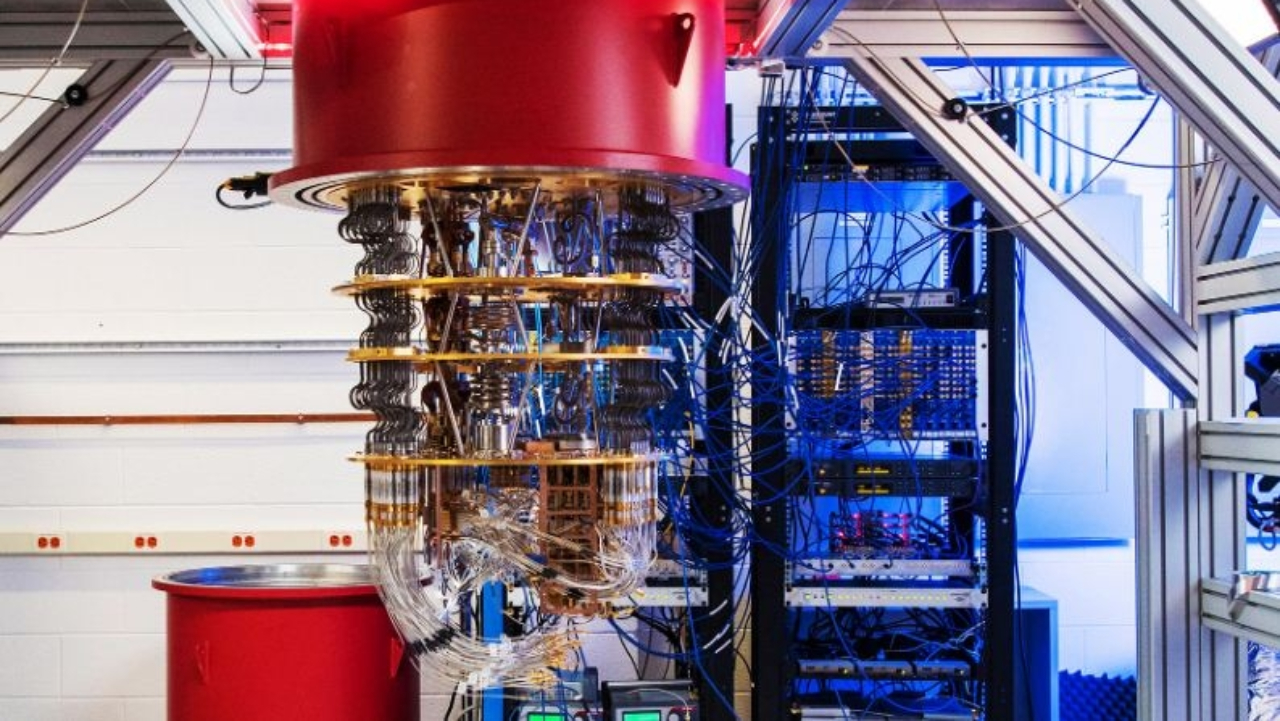
The quantum computer has been talked about for a long time. When will it be commercially available?
Probably never, as the technical challenges involved in building such a machine are enormous.
In a quantum processor, information is stored on particles, which become qubits. However, these are very unstable and generate a lot of errors. To function properly, the machine needs to be completely protected from any vibrations, electric or magnetic fields and light sources. It must also be placed in a super freezer, since its ideal operating temperature is close to absolute zero (-273°C). It is only at these temperatures that the particles remain still enough to be “manipulated”.
Despite these difficulties, all the major powers are spending billions on quantum research and development. The subject is very fashionable. The giants of technology (IBM, Intel, Honeywell etc.) and online commerce (Amazon, Alibaba) are also investing in this area.
Rather than a complete computer (or even a laptop, which is pure science fiction for the time being), it is more reasonable to imagine quantum processors installed in ad hoc premises that could be consulted remotely via the internet. The supposed computing power of such machines would be of no help for most tasks we perform on our computers every day, such as typing, sending an email, editing a photo, making a video or surfing the web. It would only really be useful for very complex and specific operations.
Edited by Sabrina Weiss. Translated from French by Catherine Hickley.
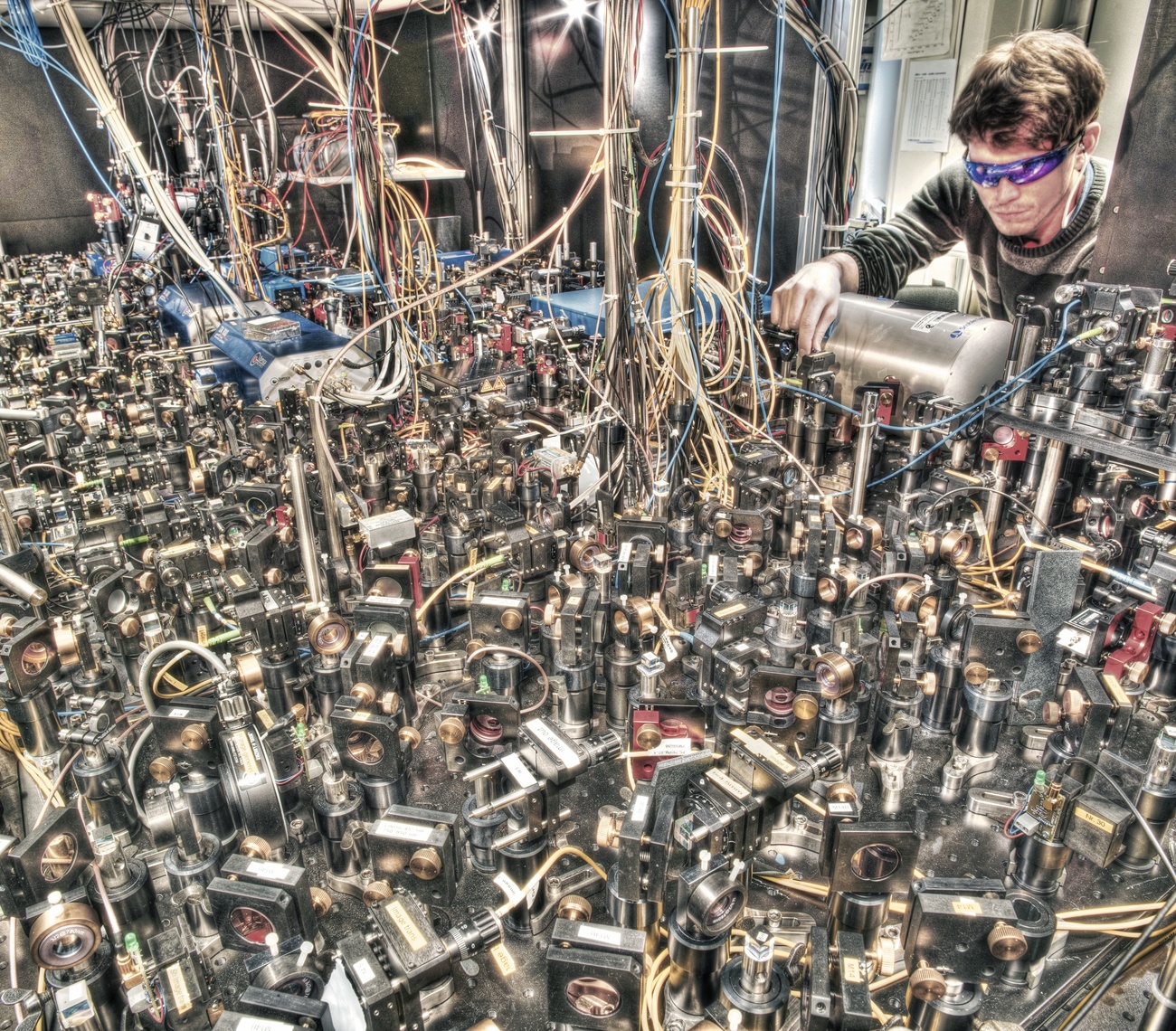
More
How science and diplomacy inform each other
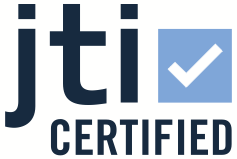
In compliance with the JTI standards
More: SWI swissinfo.ch certified by the Journalism Trust Initiative
You can find an overview of ongoing debates with our journalists here . Please join us!
If you want to start a conversation about a topic raised in this article or want to report factual errors, email us at english@swissinfo.ch.